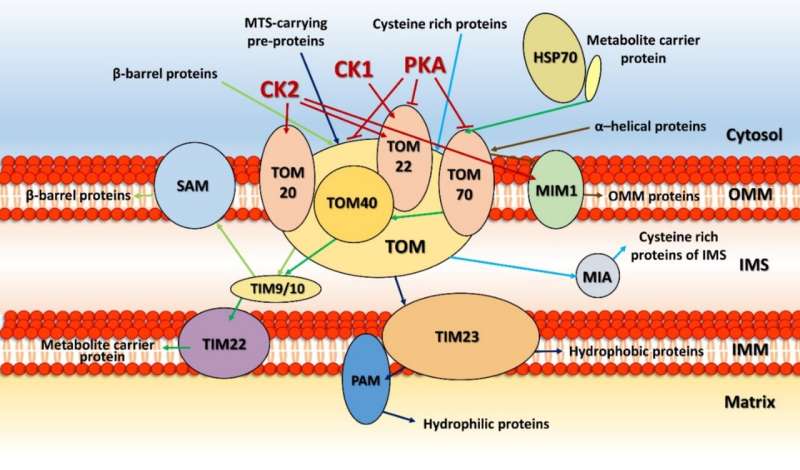
The human cellular kinome contains over 500 kinases, accounting for almost 2% of all our genes. It is currently impossible to gauge the phosphorylation status, or even phosphorylation potential, of the entire proteome of any cell. Mitochondria, on the other hand, use just 25 kinases. Moreover, their entire proteome contains only 1,136 proteins, at least according to the latest version of the Mitocarta Database.
As mitochondria retain their own versions of many of the so-called central dogmatic and other key metabolic processes found in the cytosol and nucleus, they represent a more tractable microcosm of their host cell. In light of these truths, the time is ripe to ask: Can we now fully comprehend the scope of all interactions to be found in the mitochondrial kinome?
In a recent review in the journal Life, researchers define the current state of the art in mitochondrial protein phosphorylation. Proteomic studies have revealed that ~40% of the organellar proteome is post-translationally modified by kinases. The acid test for whether increasingly fine-grained remuneration of the mitochondrial phosphorylation state provides actionable knowledge—or collapses the fort under a morass of tangled data—is what it can tell us about health and disease. In other words, what does the mitochondrial kinome reveal right now, as far as potentially helping to solve cancer, diabetes, heart dysfunction, aging and neurodegenerative or rare diseases?
Right out of the gate, there are several things we want to know. The location of the kinase, whether it is in the matrix, in the inner or outer membrane, or in the intermembrane space, reveals what partners it might be expected to act on. Usually, one can determine the mode of entry into mitochondria by looking at its particular mitochondrial localization sequence (MLS), which targets them to specific transporters on different membranes. Unfortunately, most mitochondrial kinases don't even have any MLS to speak of, and they must therefore find more creative means of gaining access to mitochondria.
For example, some kinases hijack proteins embedded in the outer membrane and use them as a scaffold to eventually get through. Cytosolic Jun N-terminal kinase interacts in this way with membrane-bound Sab protein, which itself is a substrate for p38 MAPK. Similarly, the cAMP-dependent protein kinase A (PKA) can be docked at the outer membrane by the A-kinase anchoring proteins AKAP1. The AKAP1 protein is also phosphorylated by AMPK, which also has two other mitochondrial substrates, ACC2 (acetyl-CoA carboxylase 2), and the mitochondrial fission factor (MFF). The only kinases so far found to possess true MLS sequences are PINK1, PAK5 (p21-activated kinase 5) and creatine kinase.
Kinases can control the mitochondrial import process itself in two important ways. They either phosphorylate the mitochondrial signaling pre-sequences of proteins before they get imported, or they directly modify import proteins of the TIM-TOM translocators, PAM (protein import motor) complex, or SAM (sorting and assembly machinery). The outer membrane contains other serine/threonine-specific kinases in addition to those just mentioned above, namely, ERK1/2, CDK5, LRRK2, mTOR, CAMK, CDK11, casein kinases 1 and 2 (CK1, CK2) and three isoforms of protein kinase C (PKC α, PKC δ and PKC ε).
Steroid hormone synthesis in mitochondria is rate-limited by transfer of cholesterol from the outer to inner membrane. Upon hormonal stimulation, steroidogenic acute regulatory protein (StAR) moves from the inner or outer membrane upon mitochondrial fusion, where it is activated by the dual actions of PKA and ERK1/2. StAR's consensus serine phosphorylation sites are conserved across all eukaryotes. Another key phosphorylation-controlled metabolic process unfolds at the pyruvate dehydrogenase complex (PDC) on the mitochondrial inner membrane. The PDC is one of the largest and most complex multi-enzyme systems known and its activity is controlled by multiple reversible phosphorylations.
Perhaps the most significant kinase-controlled mitochondrial function is oxidative phosphorylation. The authors present an intriguing analysis of the global phosphorylation landscape for all five complexes of the respiratory chain. One may control electron throughput in the respiratory chain via activation of proteases that control how many complexes are active. These same proteases also control protein import as they cleave the MLS signals to activate and fold the proteins upon entry.
An increasing popular approach to understanding kinase dynamics is simulation. Numerical approaches can accurately model not only kinases and accessible phosphorylation sites, but also the consumption and generation of phosphate groups available from a local diffusible pool. However, it is hard to ignore the fact that chemiosmotic phosphorylation, the bread and butter of the complex V ATPase, would tend to dominate phosphate dynamics in the tight confines of mitochondria, swamping the contributions of substrate-level phosphorylations to small molecules and proteins and making accurate simulation difficult.
To get some idea of the scale of background ADP-to-ATP phosphorylation we would be talking about here, consider that the bulk of the oxygen we breathe is converted into H2O at complex IV. This occurs through the addition of two electrons and two H+ ions to each oxygen, making four electrons per O2 molecule. For the entire human body, the total electron current from complex IV alone would be upwards of 100 amps. One might assume the energy requirements for a 70kg person might be somewhere in the neighborhood of 10,000 kJ. If the standard free energy of hydrolysis, ΔG0', of MgATP is ~30.5 kJ mol−1, we would be talking about an individual demand of about 328 mol or 165 kg of ATP per day. As the body contains only about 50 g of ATP, each ATP molecule would be synthesized and hydrolysed over 3000 times each day.
The full extent of mitochondrial kinome activites is rapidly coming into view. Nucleiods and mitoribosomes are now understood to be important kinase targets for controlling DNA maintenance and repair, transcription and protein synthesis. Activities on a slightly more macroscopic scale, including fusion, fission, mitophagy and apoptosis, are all at least partially controlled by phopshorylation. Translating these findings into direct medical benefit, as has already been done for specific cytosolic kinase activites, is now within our grasp.
© 2021 Science X Network
Citation: Complete characterization of the full mitochondrial kinome (2021, February 8) retrieved 8 February 2021 from https://ift.tt/3jFFdAt
This document is subject to copyright. Apart from any fair dealing for the purpose of private study or research, no part may be reproduced without the written permission. The content is provided for information purposes only.
"complete" - Google News
February 08, 2021 at 09:10PM
https://ift.tt/3jFFdAt
Complete characterization of the full mitochondrial kinome - Phys.org
"complete" - Google News
https://ift.tt/2Fvz4Dj
https://ift.tt/2YsogAP
Bagikan Berita Ini
0 Response to "Complete characterization of the full mitochondrial kinome - Phys.org"
Post a Comment